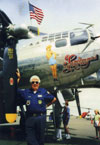
Oceanographer Bob Stevenson, on tour with the B-17G, Sentimental Journey of the Confederate Air Force, in Pocatello, Idaho, August 1998. Dr. Stevenson was an aerial navigator in World War II and flew from England in B-17s with the U.S. 8th Air Force. |
|
The Levitus, et al. Study
In their paper, Syd Levitus and colleagues describe their efforts to quantify the heat content of the world ocean from the surface through a depth of 3,000 meters, over the years from 1948 through 1998. They calculate that there was an increase of about 2 x 1023 joules from 1955 to 1995, which computes into a mean warming of the ocean (from surface to 3,000 meters depth) of 0.06°C. The increased heat content of the global ocean, they note, indicates a warming rate of 0.3 watts/m2 over the Earths surface.
The authors conclude that substantial changes in heat content took place in the 300- to 1,000-meter layers of each ocean, and at depths even greater than 1,000 meters in the North Atlantic. From these changes, they determined that in the upper layer (0-300 meters), the mean temperature of the global ocean had increased by 0.31°C during the last half century.
Explaining the impetus for their study, they write: [T]he role of the ocean [is] critical to understanding the variability of the Earths climate system . . . because of the high density and specific heat of water. As a result, the world ocean could store large amounts of heat and remove this heat from direct contact with the atmosphere for long periods of time.
Furthermore, Levitus et al. argue, [T]he Earth system is not in local radiative balance, and therefore transport of heat from the tropics to the poles is required for the Earth system to be in global radiative balance.
To address these processes, Levitus and his colleagues began to accumulate historical, upper-ocean thermal data that were available in NOAAs archives. Gridded analyses of the existing data were prepared for the period of 1960-1990. They also used the World Ocean Data base to analyze temperature anomaly fields in the ocean.
Using techniques of statistical analysis that have long been in practice (Andersen 1974; Kaylor 1977; Preisendorfer and Mobley 1988), they prepared five-year running composites of all historical ocean temperatures from 1948-1996 (sic) at standard depths levels, from the surface through 3,000 meters. It was necessary, they note, to construct multi-year composites of deep-ocean data for multi-year periods, because of the lack of deep-ocean observations (Amen, Charlie).
These time series were made for each ocean basin. Both of the Pacific Ocean basins (north and south) show quasi-bidecadal changes in the upper ocean heat content, with the two basins correlated. During 1997, the Pacific reached its maximum heat content (but the time period isnt noted).
In order to place our results in perspective, the authors then report, we compared the range of upper-ocean heat content with the range of the climatological annual cycle of heat content for the Northern Hemisphere (Levitus and Antonov 1997). They determined that there is relatively little contribution to the climatological range of heat content from depths below 300 meters!
It seemed apparent, however, they write, that the decadal variability of the upper-ocean heat content in each basin is a significant percentage of the range of the annual cycle for each basin. (This is as noted in the North Pacific by Moisan and Niiler 1998; Nakamura, Lin, and Yamagata 1997; Tanimoto, Iwasaka, Hanawa, and Tobe 1993; and Watanabi and Mizumo 1994.)
The Levitus group looked particularly at the data for the deep waters of the North Atlantic, choosing to address a depth of 1,750 meters. They learned that that ocean had warmed in the period between 1955 and 1974, and again between 1974 and 1988. The warming was not uniform, horizontally or vertically, but they determined that the changes were not small, and could have made appreciable contributions to the Earths heat balance on decadal time scales. Maximum heat storage was at depths greater than 300 meters.
So, we have the added knowledge that the heat content of the North Atlantic is substantial at depths below 300 meters. The temporal variability of the South Atlantic differs from that in the North, the latter responding to the deep ocean convective processes that occur. Regarding the World Ocean, they reported: The Pacific and Atlantic have been warming since the 1950s, and the Indian since the 1960s. The delay in the Indian Ocean may be caused by the sparsity of data before 1960.
The NOAA Conclusions
The bottom line conclusions claimed by the NOAA study are these:
(1) The world ocean has exhibited coherent changes of heat content during the past 50 years, resulting in a net warming.
(2) There is no determination whether the observed warming is caused by natural variability or anthropogenic (man-induced) forcing.
(3) The warming supports the contentions of global-climate modellers that the planetary radiative disequilibrium, for the period of 1979 to 1996, may be the result of excess heat accumulating in the ocean.
(4) Sea-surface temperatures have had two distinct warming periods over the past century; from 1920 to 1940, then a cooling period until the second warming began in the 1970s.
(5) In each period of warming, an increase in the oceans heat content preceded the observed warming of the sea-surface temperatures. The NOAA scientists concluded that it could be the result of natural variability, or anthropogenic effects, or more likely both.
(6) It was speculated that the extreme warmth of the world ocean during the mid-1990s was caused by (a) the multi-decadal warming of the Atlantic and Indian oceans, and (b) a positive polarity in a possible bidecadal oscillation of the Pacific Ocean heat content.
(7) And a final point, regarding the large change in Atlantic heat storage at depths exceeding 300 meters: The convection in the Labrador Sea, by mixing the ocean through a 2,000-meter-deep water column, may keep sea-surface temperature changes relatively small, despite a large heat flux from ocean to atmosphere. Such convection must be addressed, especially when anthropogenic forcing is being considered.
So, How Does This Play in Hanalei?
(Considering that Hanalei, Hawaii is just down the hill from where I write, I thought Id inject a little local color into my comments.) It sometimes seems as if Im living in a time-warp in which some people, and scientists, are unaware that rational life existed before their birthor before they got out of the sixth grade. Yet, we marine scientists did not enter the second half of the 20th century without a fair bit of understanding of the thermal ocean.
For example, Prof. Hubert H. Lamb, the premier European climatologist of the 20th century,1 wrote in 1977 that there has been a general warming of sea temperatures, by 0.5-1.0°C, from 1880 to 1965, defined from widely scattered points around the oceans of the world. Lamb went on to say that This general warming is known from the Gulf of Alaska, the eastern Pacific Ocean, the western Indian Ocean, the eastern and northern North Atlantic Ocean, and the tropics of both the Atlantic and Indian oceans.
Within those 85 years, Professor Lamb noted that there were minima in the periods of 1915-1925 and again between 1940 and 1950meaning that the rate of temperature rise went to zero, but temperatures did not decline to levels lower than they had already reached. For the Atlantic Ocean, 55°N to 40°S, the waters were cooler by 0.8°C to 1.0°C in 1780-1850 than in 1950. Now, the temperatures that Professor Lamb provides were certainly not taken as precisely, nor were they as many as we have acquired in the past half century. But, their existence is not trivial.
Sources of 20th Century Ocean Temperatures
I learned to deploy Nansen water bottles and reversing thermometers for deep-sea sampling in 1949. I spent the rest of the subsequent decade seagoing, for the most. I cant remember how many bottle casts I made, or how many bathythermographs I deployed. There had to be thousands in the waters off coastal California. Other students and post-docs were doing the same farther offshore in the eastern Pacific, from the E.W. Scripps. In the westernmost Atlantic, a similar cadre worked from the Atlantis.
In the 1960s, more ships were out at sea: from Fisheries Laboratories, U.S. Coast and Geodetic Survey (now NOAA), and research institutions at Scripps (La Jolla, Calif.), Woods Hole (Massachusetts), Miami, and Texas A&M (in the Gulf of Mexico). The British sailed the new Discovery, the Germans the new Meteor, and there were small ships sailing from Denmark, Japan, and France. Many cruises were dedicated to the geophysics of the sea floor, where deep-ocean casts for water and temperatures were few and far between.
Surface water samples were taken routinely, however, with buckets from the deck and the ships engine-water intake valve. Most of the thermometers were calibrated into 1/4-degrees Fahrenheit. They came from the U.S. Navy. Galvanized iron buckets were preferred, mainly because they lasted longer than the wood and canvas. But, they had the disadvantage of cooling quickly in the winds, so that the temperature readings needed to be taken quickly. I would guess that any bucket-temperature measurement that was closer to the actual temperature by better than 0.5° was an accident, or a good guess. But then, no one ever knew whether or not it was good or bad. Everyone always considered whatever reading was made to be precise, and they still do today. The archived data used by Levitus, and a plethora of other oceanographers, were taken by me, and a whole cadre of students, post-docs, and seagoing technicians around the world. Those of us who obtained the data, are not going to be snowed by the claims of the great precision of historical data found stored in some musty archives.
I am more than a bit curious about the great scavenger hunt by the folks at NOAA/NESDIS (National Environmental Satellite Data Information System). In 1970, with the advent of the International Decade of Ocean Exploration, all institutions under contract with any governmental agency, and all governmental agencies, were required to send their data to the National Ocean Data Center; and that included data gathered before 1970. They were permitted a certain lead time to do thatabout five years, as I recall. Those data were made more accessible by the GISST (Global Ice and Sea Surface Temperature) data set, put together by Folland and Powell in 1994 from the Hadley Center in England, and the bathythermograph data sets (BTs) put together by the Scripps Institution. Nearly all of the latter BTs were deployed through programs coordinated with NOAA, so Im guessing that no one at NOAA had to look far for those data.
Some Basics of Marine Climatology
I wrote my first paper on the oceans influence on climate in 1958. The next year I was in England, working for the Navy to learn whether or not micro-climates, as we called them, along shores of the North Sea were determined by the adjacent coastal ocean. During the year, I visited all of the marine laboratories and research centers in western Europe.
It was in Germany, at the Seewetteramt (Marine Branch of the German Meteorological Office), where I met and began to work with two outstanding marine climatologists, Martin Rodewald, and Hans Markgraf, and the director, Dr. Hans U. Roll, the premier marine meteorologist of the time. They were looking at much larger areas than Inamely, the North Atlantic and the polar seasand how they influenced the climate and weather over northwest Europe. It was a great education for me. I learned the processes by which the ocean and atmosphere work together.
The basics of these interactions start where oceans and atmosphere meet. More than 70 percent of the Earths surface is covered by oceans, seas, and lakes, and another 5 percent is covered by glaciers and ice caps. Just more than two thirds of this water area is in the Southern Hemisphere, and the oceans are 4 to 5 kilometers deep.
The atmosphere cannot warm until the underlying surface warms first. The lower atmosphere is transparent to direct solar radiation, preventing it from being significantly warmed by sunlight alone. The surface atmosphere thus gets its warmth in three ways: from direct contact with the oceans; from infrared radiation off the ocean surface; and, from the removal of latent heat from the ocean by evaporation. Consequently, the temperature of the lower atmosphere is largely determined by the temperature of the ocean.
Inland locations are less restrained by the oceans, so the surface air experiences a wider temperature range than it does over the oceans. Land cannot store heat for long, which is why hot days are quickly followed by cold nights in desert regions. For most of the Earth, however, the more dominant ocean temperatures fix the air temperature.
This happens through several means:
(1) The oceans transport heat around the globe via massive currents which sweep grandly through the various ocean basins. As a result, the tropics are cooler than they would be otherwise, and the lands of the high latitudes are warmer. The global circulation of heat in the oceans moderates the air temperatures around the whole world.
(2) Because of the high density/specific heat of sea water, the entire heat in the overlying atmosphere can be contained in the top two meters of the oceans. This enormous storage capacity enables the oceans to buffer any major deviations in temperature, moderating both heat and cold waves alike.
(3) Evaporation is constantly taking place at the surface of the seas. It is greatest in the tropics and weakest near the polar regions. The effect of evaporation is to cool the oceans and, thereby, the surface atmosphere.
How the Oceans Get Warm
Warming the ocean is not a simple matter, not like heating a small glass of water. The first thing to remember is that the ocean is not warmed by the overlying air.
Lets begin with radiant energy from two sources: sunlight, and infrared radiation, the latter emitted from the greenhouse gases (water vapor, carbon dioxide, methane, and various others) in the lower atmosphere. Sunlight penetrates the water surface readily, and directly heats the ocean up to a certain depth. Around 3 percent of the radiation from the Sun reaches a depth of about 100 meters.
The top layer of the ocean to that depth warms up easily under sunlight. Below 100 meters, however, little radiant energy remains. The ocean becomes progressively darker and colder as the depth increases. (It is typical for the ocean temperature in Hawaii to be 26°C (78°F) at the surface, and 15°C (59°F) at a depth of 150 meters.
The infrared radiation penetrates but a few millimeters into the ocean. This means that the greenhouse radiation from the atmosphere affects only the top few millimeters of the ocean. Water just a few centimeters deep receives none of the direct effect of the infrared thermal energy from the atmosphere! Further, it is in those top few millimeters in which evaporation takes places. So whatever infrared energy may reach the ocean as a result of the greenhouse effect is soon dissipated.
The concept proposed in some predictive models is that any anomalous heat in the mixed layer of the ocean (the upper 100 meters) might be lost to the deep ocean. There have been a number of studies in which this process has been addressed (Nakamura 1997; Tanimoto 1993; Trenberth 1994; Watanabi 1994; and White 1998). It is clear that solar-related variations in mixed-layer temperatures penetrate to between 80 to 160 meters, the average depth of the main pycnocline (density discontinuity) in the global ocean. Below these depths, temperature fluctuations become uncorrelated with solar signals, deeper penetration being restrained by the stratified barrier of the pycnocline.
Consequently, anomalous heat associated with changing solar irradiance is stored in the upper 100 meters. The heat balance is maintained by heat loss to the atmosphere, not to the deep ocean. |
Return to top |
|
What about Thermohaline Circulation?
The fact that the surface ocean can become denser than the underlying waters, thereby sinking to depths of density equilibrium, has been discussed since surveys of the physical ocean began in the second half of the 19th century. Certainly the concept was known before HMS Challenger sailed, in 1873, on its famous expedition. One of the multitude of suggestions made by members of the Royal Society at that time was to investigate the over-turning of surface waters caused by density differences.
Thermohaline circulation is responsible for the formation of the bottom-water masses in the worlds oceans: the North Atlantic Deep Water (NADW) originates basically in the region of the Labrador Sea; the Weddell Sea is the source of the deep-water in the circumpolar Southern Ocean; and the Pacific Deep Water originates in the Ross Sea. In many other places in the oceans, and seas, as well, surface waters are carried into the depths by thermohaline circulation.
So, it is not surprising that those modellers who need to get warm surface waters to move into the depths of the oceans, and remain sequestered there for long periods of time, would turn to the physical mechanism of this vertical circulation system. Their hope (claim) is that there can be occasions when salinity, rather than temperature, is the prime determining factor in the density of the surface waters. Then, warm water, made dense by an increase in the seas salt content, would sink.
It does not happen!
The primary physical factor in determining the density of sea water is the temperature (Sverdrup, Johnson, and Fleming, 1943). In the open ocean, top or bottom, salinity differences are measured in a few parts per thousand. Thermohaline circulation takes place where the surface waters become colder than the waters beneath. The large vertical movements occur in polar seas, where accelerated radiation makes the surface waters greatly colder than the deeper waters.
In these waters, surface water temperatures are about -1.9°C, the normal salinity of the water keeping it from freezing into ice. The deep waters, being warmer than such surface waters, rise to the surface, as the upper layers sink slowly into the dark ocean depths. Because only very cold surface water is able to sink, it is simple to understand that the deep ocean can never warm up, regardless of how warm the surface ocean around the world may become. No deep lying thermal lag is going to take place. It is clear that therell be no Phoenix rising as a haunting specter.
The Big, Deep-Blue Sea
To one extent or another, Ive been involved with the relationships of the oceans on climates, and vice versa for the past 50 years. It was when I became Secretary General of IAPSO, in 1987, to work closely with our sister associations of Meteorology (IAMAP), Hydrology (IAHS), and Volcanology (IAVCEI), all within our mother union International Union of Geodesy and Geophysics (IUGG) that I first had to face the claim coming from the United Nations Environment Program and World Meteorological Organization that global warming of the atmosphere was in full swing, induced by the over-enthusiasm of mankind to travel, keep warm, and feed themselves. By their desire to enhance their lives, human beings were increasing, untenably, the CO2 content of the Earths greenhouse. I was frankly surprised by this claim, and believed it not one whit.
As an oceanographer, Id been around the world, once or twice, and I was rather convinced that I knew the factors that influenced the Earths climate. The oceans, by virtue of their enormous density and heat-storage capacity, are the dominant influence on our climate. It is the heat budget and the energy that flows into and out of the oceans that basically determines the mean temperature of the global atmosphere. These interactions, plus evaporation, are quite capable of cancelling the slight effect of man-produced CO2.
In 1991, when the IUGG and its associations met in Vienna for their General Assembly, the presidents and the secretaries-general of the four associations Ive mentioned, discussed the program we would propose to forward to the International Commission of Scientific Unions (ICSU) for consideration at the 1992 Rio de Janeiro Conference. We all decided not to prepare any programs!
In our joint statement, which I paraphrase here, we noted that To single out one variable, namely radiation through the atmosphere and the associated greenhouse effect, as being the primary driving force of atmospheric and oceanic climate, is a simplistic and absurd way to view the complex interaction of forces between the land, ocean, atmosphere, and outer space.
Furthermore, we stated, climate modelling has been concentrated on the atmosphere with only a primitive representation of the ocean. Actually, some of the early models depict the oceans as nearly stagnant. The logical approach would have been to model the oceans first (there were some reasonable ocean models at the time), then adding the atmospheric factors.
Well, no one in ICSU nor the United Nations Environment Program/World Meteorological Organization was ecstatic about our suggestion. Rather, they simply proceeded to evolve climate models from early weather models. That has imposed an entirely atmospheric perspective on processes which are actually heavily dominated by the ocean.
So, where does the NOAA paper fit?
I was rather eager to read the article by Syd Levitus, and his colleagues. I was somewhat put-off by the headlines about missing warming, but I figured that was just the usual hype by the media.
Yet, here I sit in the middle of the Pacific Ocean, surrounded by papers (peer-reviewed, I guess I should add) which conclude:
(1) For the past two decades at least, and possibly for the past seven decades, the Earths true surface air temperature has likely experienced no net change;
(2) there should have been a sizable CO2-induced increase in atmospheric radiative forcing during that time, but there wasnt. That must mean that a suite of compensatory feedbacks overwhelmed the greenhouse impetus for warming; implying, therefore,
(3) that the planet will not warm from any man-produced increases in CO2; indicating
(4) any increases in temperature will likely fit the global trend of +0.048°C/decade, that is, about 0.5°C this century the rate of warming that has existed since the Little Ice Age, centered around 1750 in Europe, South America, and China; suggesting
(5) that the heat storage in the upper ocean takes place in the upper 100 meters, and the magnitude provides a rise in temperature at those depths of 0.5°C in the past 50 years (in those parts of the ocean for which we have data);
(6) this global warming (and cooling) of the ocean occurs on biennial, ENSO, decadal and interdecadal period scales; thence,
(7) the ocean thermal changes on centennial-period scales, which appear as the warming trend through the past 50 to 100 years, can be explained by means of intrinsic internal modes of the Earth going through their normal cycle of warming and cooling, independent of both radiative and anthropogenic influences.
I guess what Im really wondering is Why did Syd Levitus, and his associates, write their paper in the first place?
Robert E. Stevenson, an oceanography consultant based in Hawaii, trains the NASA astronauts in oceanography and marine meteorology. He was Secretary General of the International Association for the Physical Science of the Oceans from 1987 to 1995, and worked as an oceanographer for the U.S. Office of Naval Research for 20 years. A member of the scientific advisory board of 21st Century, he is the author of more than 100 articles and several books, including the most widely used textbook on the natural sciences.
NOTES
1. Hubert H. Lamb was Britains most outstanding meteorologist through and after World War II. What the British and American air forces accomplished in weather forecasts was the result of his work. He is the author of numerous papers and several exhaustive studies in historical climatology.
REFERENCES
N. Andersen, 1974. On the calculation of filter coefficients for maximum entropy spectral analysis, Geophysics, Vol. 39.
J.I. Antonov, et.al., 1998. World Ocean Data Atlas, Atlantic Ocean temperature fields, Vol. 1, NOAA Atlas, NESDIS 27.
J. Bjerknes, 1966. A possible response of the atmospheric Hadley circulation to equatorial anomalies of ocean temperatures, Tellus, Vol. 18.
C. Deser, and M. Blackman, 1993. Surface climate variation over the North Atlantic Ocean during winter: 1900-1999, J. Clim., Vol. 6.
C. Deser, M.A. Alexander, and M.S. Timlin, 1993. Upper-ocean thermal variations in the North Pacific during 1970-1991, J. Clim., Vol. 9.
D.K. Folland, D.E. Parker, and F.E. Kates, 1984. Worldwide marine temperature fluctuations 1856-1981, Nature, Vol. 310.
D.K. Folland and D.P. Powell, 1994.The Standard GISST Data Sets: Version 1 and 2, Clim. Res. Tech. Note 56, Bracknell, England: Hadley Center.
P. Foukal, and J. Lean, 1990. An empirical study of total solar irradiance variation between 1874-1988, Science, Vol. 247.
J.G. Graham, 2000. The formation and propagation of North Atlantic heat content anomalies, Eos, Trans. AGU, Vol. 80.
N.E. Graham, W.B. White, and A. Pares-Sierra, 1990. Low frequency ocean-atmosphere interactions in the tropical Pacific, in Air-Sea Interactions in the Tropical Western Pacific, In Air Sea Interactions in the Tropical Western Pacific, Eds. C. Jiping and J. Young (Beijing: China Ocean Press).
S.L. Howard, and R. D. Muench, 2000. Upper ocean stability and water mass formation northwest Weddell Sea winter, Eos, Trans. AGU, Vol. 80.
Z. Jaworowski, 1996. Greenhouse Gases in Polar Ice-Artifacts or Atmospheric Reality?, Umseit und Chemie, (Ulm: Gesellschaft Deutscher Chemiker, Oct. 7-10).
Z. Jaworowski, T.V. Segelstad, and V. Hisxdal, 1992. Atmospheric CO2 and Global Warming, Oslo Norsk Polarinstitutt, Meddeleiser, Vol. 119.
R.E. Kaylor, 1977. Filtering and decimation of digital time series, Tech. Rep. Note BN 850, Inst. Phys. Sci. and Tech. (College Park, Md.: Univ. of Maryland).
L.D. Keigwin, 1996. The Little Ice Age and Medieval Warm Period in the Sargasso Sea, Science, Vol. 274.
K.A. Kelly, 2000. Interannual-to-decadal variations in the upper ocean heat budget in the Western North Pacific, Eos, Trans. AGU, Vol. 281.
Y. Kushnir, 1994. Interdecadal variations in the North Atlantic sea surface temperature and associated atmospheric conditions, J. Clim., Vol. 11.
M. Latif, and T.P. Barnett, 1994. Causes of decadal climate variability over the North Pacific and North America, Science, Vol. 266.
J.L. Lean, O.R. White, and A. Skumanich, 1995. The solar ultraviolet irradiance in the Maunder Minimum, Glob. Biogeochem. Cycles, Vol. 28.
S. Levitus, J.L. Antonov, T.P. Boyer, and Cathy Stephens, 2000. Warming of the world ocean, Science, Vol. 287.
M.E. Mann, J. Park, and R.S. Bradley, 1995. Global interdecadal and century-scale climate oscillations during the past 5 centuries, Nature, Vol. 378.
A.J. Miller, D.R. Cayan, and W.B. White, 1998. A decadal change in the North Pacific thermocline and gyre-scale circulation, J. Phys. Ocean., Vol. 27.
S. Minobe, 2000. Pacific Pentadecadal Oscillation, Eos, Trans. AGU. Vol. 218.
J.R. Moisan, and P.P. Niiler, 1998. The seasonal heat budget North Pacific; Net heat flux and storage rates (1950-1990), J. Phys. Ocean., Vol. 28.
______, 1998. Decadal climate variability in the North Pacific during recent decades, Bull. Am. Met. Soc., Vol. 78.
N.E. Newell, R.E. Newell, J. Hsuing, and W. Zhongxiang, 1989. Global marine temperature variation; the solar magnetic cycle, Geophys. Res. Lett., Vol. 16.
D.E. Parker, P.D. Jones, C.K. Folland, and A. Bevan, 1994. Interdecadal changes of surface temperature since the late nineteenth century, J. Geophys. Res. Vol. 99.
E.S. Posmetier, W.H. Soon, and S.L. Baliunas, 1998. Correlations from Solar Irradiance," in Global WarmingThe Continuing Debate, European Science and Environment Forum.
R.W. Preisendorfer, and C.D. Mobley, 1988. Principal Component Analysis in Meteorology and Oceanography (New York: Elsevier).
A. Sarkisyan, and S. Levitus, 2000. Ocean Climate Characteristics by Amalgamating WOCE-Levitus Hydrographic Data, Eos, Trans. AGU, Vol. 80.
I.D. Schroeder, B.L. Lipphardt, T.C. Royer, A.D. Kirwan, and C.E. Grosch, 2000. Normal mode analysis of North Pacific SSTs, Eos, Trans. AGU, Vol. 80.
T.V. Segelstad, 1996. Carbon Isotope Mass Balance of Atmospheric CO2, in The Global Warming Debate, Ed. J. Emsley, (London).
R.E. Stevenson, 1962. Climatic amelioration in Southern California, 1955-1960, J. Applied Met., Vol. 1.
______, 1964. The influence of a ship on the surrounding air and water temperatures, J. Applied Met., Vol. 3.
______, 1996. An oceanographer looks at the non-science of global warming, 21st Century, Vol. 9.
R.E. Stevenson, and R.S. Armstrong, 1965. Heat loss from the surface waters, northwest Gulf of Mexico from Hurricane Carla, Geofys. International, Vol. 5.
H. Svensmark, and E. Friis-Christensen, 1997. Cloud suppression by solar winds, J. Atmos. Sol-Terrest. Phys, Vol. 59.
H.U. Sverdrup, M.W. Johnson, and R.H. Fleming, 1942. The Oceans: Their Physics, Chemistry and General Biology (New York: Prentice Hall).
Y. Tanimoto, N. Iwasaka, K. Kananwa, and Y. Tobe, 1993. ENSO signals in global upper ocean temperature, J. Clim., Vol. 6.
K.E. Trenberth, and J.W. Hurrell, 1994. Decadal atmospheric-ocean variations in the Pacific, Clim. Dyn., Vol. 9.
F. Vauclair, and Y. Du-Penhoat, 2000. Interannual Variability in the Tropical Atlantic Ocean between 1979 and 1999 from a Gridded Data Set, Eos, Trans. AGU, Vol. 80.
T. Watanabe, and K. Mizuno, 1994. Decadal changes in the thermal structure of the North Pacific, Int. WOCE Newsl. Vol. 15.
W.B. White, R. L. Bernstein, G. McNally, R. Dickson, and S. Pazan, 1980. The thermocline response to the transient atmospheric forcing in the interior mid-latitude Pacific Ocean, J. Phys. Ocean., Vol. 10.
W.B. White, and D.R. Cayan, 1998. Quasi-periodicity and global symmetries in interdecadal upper ocean temperature variability, J. Geophys., Vol. 103.
W.B. White, J. Lean, D.R. Cayan, and M.D. Dettinger, 1997. Response of global upper ocean temperature to changing solar irradiance, J. Geophys. Res., Vol. 102.
T. Wigley, and P.M. Kelly, 1992. Solar cycle length, greenhouse forcing and global climate, Nature, Vol. 360 |